abstract 摘要
此文提出了玉米秸秆产生的一个典型的170经DAM3干磨乙醇厂供热供电。玉米乙醇厂需要5.6兆瓦的电力和52.3兆瓦的热过程,创造了高达140的GG每年秸秆的需求。玉米秸秆供应系统由采集、预处理、运输和现场燃料储存和制备生产乙醇厂热电。整个供水系统经济学使用集成的生物质供应物流进行了分析(IBSAL)仿真模型。玉米秸秆是三格式交付(方包,干排骨和颗粒)的热电联合厂。73美元用于包交付1毫克计算成本的生物质准备被烧毁,86美元和84美元为丸1毫克1毫克现场切量。三格式的秸秆供应系统中,交付成本颗粒生物量最高,由于制粒成本高。在各种形式的生物质颗粒散装运输可以在我们提供一个有前途的未来的生物质供应物流系统,如果造粒和运输成本最小化。Supply of corn stover to produce heat and power for a typical 170 dam3 dry mill ethanol plant is proposed. The corn ethanol plant requires 5.6 MW of electricity and 52.3 MW of process heat, which creates the annual stover demand of as much as 140 Gg. The corn stover supply system consists of collection, pre-processing, transportation and on-site fuel storage and preparation to produce heat and power for the ethanol plant. Economics of the entire supply system was conducted using the Integrated Biomass Supply Analysis and Logistics (IBSAL) simulation model. Corn stover was delivered in three formats (square bales, dry chops and pellets) to the combined heat and power plant. Delivered cost of biomass ready to be burned was calculated at 73 $ Mg 1 for bales, 86 $ Mg 1 for pellets and 84$ Mg 1 for field chopped biomass. Among the three formats of stover supply systems, delivered cost of pelleted biomass was the highest due to high pelleting cost. Bulk transport of biomass in the form of chops and pellets can provide a promising future biomass supply logistic system in the US, if the costs of pelleting and transport are minimized.
1. Introduction 介绍
DOI:10.1016 / j.biombioe.2009.10.001Ethanol plants in the US use corn grain as the feedstock for producing nearly 18 hm3 of ethanol [1]. Most of the ethanol produced is blended with gasoline. The ethanol represents roughly 1.5% of the current annual volume of petroleum consumption of 1.15 km3 [2]. National plans call for increasing ethanol production to levels that would offset at least 30% of the annual transportation fuel volume within the next 20– 30 years. It is forecasted that the current and projected increase in corn grain may support starch-based ethanol production up to 75 hm3 of ethanol. Lignocellulosic biomass feedstock can further support ethanol production beyond the75 hm3 from corn grain. Therefore, it is important to develop secure sources of biomass and supply infrastructure to support the projected growth of bioenergy. A typical ethanol plant requires 9.67 MJ of process heat and 0.288 kWh of electricity to produce 1 l of ethanol. Most of the existing ethanol plants in the US use natural gas as the source of process heat and electricity from the electric grid [1,2]. Prices for natural gas and electricity have increased in recent years making ethanol production economics less attractive. To offset this issue, some plants have or are planning to use coal [3] and that has created a negative impact on public acceptance of ethanol as a ‘‘green or clean fuel’’. The technology of producing process heat and electricity from direct 0961-9534/$ – see front matter a 2009 Elsevier Ltd. All rights reserved. doi:10.1016/j.
biomass and bioenergy 34 (2010) 75–81 combustion of biomass is now well developed [4,5]. Biomass can provide heat and power to the existing and future starch-based ethanol plants.
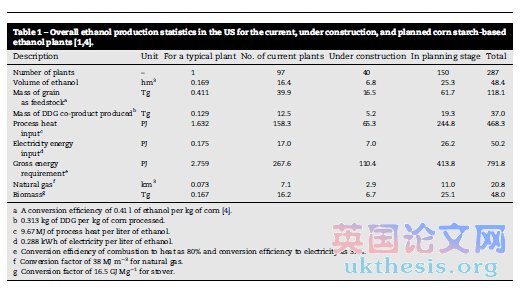
Table 1 lists the current and an estimated future number of ethanol plants in the US [1]. The list includes an estimate of the required grain (corn) as feedstock and the amount of process heat and electrical energy required to keep the plants running. An estimate of the required grain and stover and the amount of co-product, distillers dried grain (DDG) is also presented in Table 1. The last two rows are an estimate of net energy input to the plants for process heat and electricity. Assuming that 287 plants become operational in the next 5– 7 years, there will be a production of roughly 48 hm3 of ethanol from corn. Roughly 48 Tg of biomass is required to heat and power these plants if all these plants use biomass as a fuel source. Typical energy balance studies of corn ethanol show that the energy balance (defined in terms of renewable energy in the ethanol product over all non-renewable energy inputs from seed to product) ranges from 1.1 to 1.3 [4–7]. There are high energy demands in the provision of fertilizer and crop management as well as in the demand for heat and electricity in the process plant. Improvements in process plant efficiency, and the use of renewable energy inputs for electricity and heat could make the overall renewable energy balance >2. Morey et al. [4] proposed the use of DDG or corn stover for heat and electricity generation for the ethanol plants and found that there was a significant annual energy cost savings for the 170 dam3 ethanol plant. Use of DDG for power and heat generation may not be the best option due to its high nutritional value as a choice source of protein and fiber for animal feed (>80$ Mg 1). In order to compare and make corn stover competitive to the existing heating fuels, a techno-economic analysis of using biomass to supply heat and power systems should be investigated. Any biomass-based heat and power production system relies mainly on the continuous and cheap supply of biomass delivered to the plant. Our main objectives of this paper were to estimate the cost of supplying corn stover to the existing dry mill ethanol plants using the Integrated Biomass Supply Analysis and Logistics (IBSAL) model to produce heat and/or power and to calculate the cost of on-site fuel preparation for delivering it to the burner.
2. Description of IBSAL model 描述ibsal模型
The Integrated Biomass Supply Analysis and Logistics (IBSAL) is a dynamic simulation model developed by Oak Ridge National Laboratory (ORNL) to estimate the delivered biomass cost, energy input and carbon emissions for various logistic options [8]. IBSAL consists of different sub-modules for harvesting, processing, pre-processing (grinding, pelleting), storage and transportation. Model input data include: local weather data; average net yield of biomass; crop harvest progress data (including start and end dates of harvest); dry matter loss with time in storage; moisture content of plant at the time of harvest; operating parameters of equipment; and cost of machinery in dollars per hour. The model was built on the EXTENDSIM platform [9]. Main outputs of the model include: delivered cost of biomass ($ Mg 1); carbon emission (kg Mg 1) and energy consumption (GJ Mg 1). IBSAL also calculates dry matter losses of biomass using the limited data available for storing biomass bales and handling hay. Complete information about the model can be found in Sokhansanj et al. [10] and Sokhansanj and Mani [11]. The choices of particular size and operating conditions are based on three objectives: (1) the latest model of equipment that is commercially available for harvest, (2) the typical operational performance data that are available in the ASAE D497 standard [12] or from manufacturer’s literature, and Table 1 Overall ethanol production statistics in the US for the current, under construction, and planned corn starch-based ethanol plants [1,4]. Description Unit For a typical plant No. of current plants Under construction In planning stage Total Number of plants – 1 97 40 150 287 Volume of ethanol hm3 0.169 16.4 6.8 25.3 48.4 Mass of grain Tg 0.411 39.9 16.5 61.7 118.1 as feedstocka Mass of DDG co-product producedb Tg 0.129 12.5 5.2 19.3 37.0 Process heat PJ 1.632 158.3 65.3 244.8 468.3 inputc Electricity energy PJ 0.175 17.0 7.0 26.2 50.2 inputd Gross energy PJ 2.759 267.6 110.4 413.8 791.8 requiremente Natural gasf km3 0.073 7.1 2.9 11.0 20.8 Biomassg Tg 0.167 16.2 6.7 25.1 48.0 aAconversionefficiencyof0.41lofethanolperkgofcorn[4].b0.313kgofDDGperkgofcornprocessed.c9.67MJofprocessheatperliterofethanol.d0.288kWhofelectricityperliterofethanol.eConversionefficiencyofcombustiontoheatas80%andconversionefficiencytoelectricityas30%.fConversionfactorof38MJm 3fornaturalgas.gConversionfactorof16.5GJMg 1forstover. #p#分页标题#e#
biomass and bioenergy 34 (2010) 75–81 Combine grain Store on farm Square Bale Pellets Corn crop Grain Elevator Store Dry Chop Integrated Combined Heat and Power (CHP) & Ethanol Plant Corn grain Corn stover
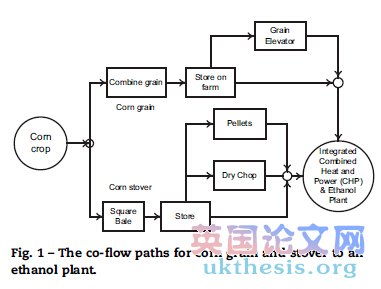
Fig. 1 – The co-flow paths for corn grain and stover to an ethanol plant. (3) limited equipment performance data published for corn stover elsewhere. Hourly costs are calculated using the procedure and data described in Sokhansanj and Turhollow [13]. The rates represent the sum of fixed and variable costs. The hourly rates for the pull-type equipment (for example, baler) are the sum of the hourly rate for the implement and the power equipment (for example, tractor).
3. Economics of corn stover supply to the ethanol plant 玉米秸秆的乙醇厂供应经济学
Almost all of the present ethanol plants in the US use corn grain as a source of feedstock. The concept is to integrate the harvest and supply of corn stover with the well-developed grain harvest and delivery system as outlined in Fig. 1. The grain is combined and stored on the farm or sent directly to a central depot (country elevator). The grain is transported to the ethanol plant either directly from the farm store or from the depot. Similar to grain that is combined and stored in the steel bins, corn stover is baled and stacked on the farm or transported to a larger storage area (depot). The supply of biomass to the ethanol plant will be in one of the three forms: (1) baled, (2) chopped/ground, or (3) pelletized. We assume a 170 dam3 ethanol plant for the analysis. The power rating of the plant is estimated to be 5.6 MW of power and 52.3 MW of process heat. The ethanol plant requires as much as 140 Gg of stover annually. All the cost data in the paper are reported based in year 2006 US dollars.
3.1. Stover collection
We define collection as operations for picking up the biomass, packaging, and transporting to a nearby site for temporary storage. The most conventional method for collecting biomass is baling [14]. Bales are in the form of either rounds or squares. Limited experience with using round bales for biomass applications indicates that round bales are not suitable for large scale biomass handling [15,16]. Because of their round shape, round bales tend to deform under static loads in a stack. Bales that are not perfectly round cannot be loaded onto trucks to form a transportable load over open roads. Therefore, square baling operation was considered in this study. The Integrated Biomass Supply Analysis and Logistics (IBSAL) model [8,11] was used to estimate the resource requirement and cost of biomass collection. We used a net stover yield of 5.7 Mg ha 1, a typical stover current yield in the US Midwest [13,17]. The collection sequence consists of shredding and baling it into large rectangular bales 1.2 1.2 2.4 m. The bales are collected using a truck mounted bale collector [18]. The Stinger transports the collected biomass to the side of the farm and stacks it in rows four bales high (4.8 m high). #p#分页标题#e#
3.2. Pre-processing
Pre-processing of biomass may consist only of grinding. Loose cut biomass has a low bulk density ranging from 50 to 120 kg m 3 depending on the particle size. The ground biomass of less than 6 mm could have a bulk density of 160 kg m 3 in the truck box [17,19]. This density may be suitable for short hauls. To process biomass bales into chopped form, a mobile shredder/grinder is used to chop the biomass. The machine is similar to an agricultural tub grinder and can be transported to the stacks. A bale is placed on the grinder/shredder; the ground biomass is then transferred to a weighing truck box using an attached belt conveyor. The cost of the biomass chopping process was estimated using the IBSAL simulation model. For longer hauls and long term storage, denser biomass in the form of pellets or cubes may be desired. The bulk density of pellets can be as high as 700 kg m 3 and cubes as high as 500 kg m 3[20]. To make pellets, the baled biomass is chopped using a tub grinder, fine ground using a hammer mill, and extruded using a pellet mill. The pellets are cooled and stored. The pellets will have a bulk density of 600–700 kg m 3. Corn Stacking/ storage Debaling/ choppingDestringingBale retrieving Dry chop storage Chop retrieving Fine grinding Pneumatic conveying Pellet storage Pellet retrieving Combined Heat & Power Plant Biomass receiving station Square bales Dry chop Pellets
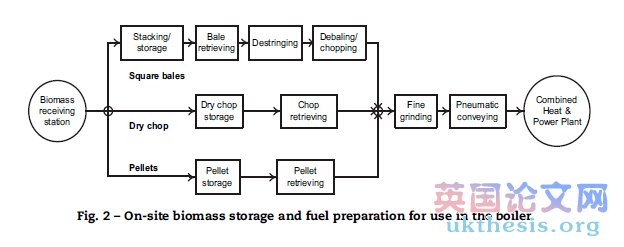
Fig. 2 – On-site biomass storage and fuel preparation for use in the boiler.
biomass and bioenergy 34 (2010) 75–81 Table 2 Completion date, cost, energy input, emissions and dry matter for a conventional baling system for corn stover at a yield of 5.7 Mg haL1 . Operationa Date completedb Mass (Mg) Cost ($ Mg 1) Energy input (MJ Mg 1) Carbon emission (kg Mg 1) Payment to producer Shredding Balingc Stacking Profit (15% of the collection costs) Overall Dec 10 Dec 13 Dec 16 141550 140359 139819 10.00 4.11 12.36 7.89 3.65 38.01 47.4 136.9 123.5 313.7 1.0 2.9 2.7 6.7 aNo.ofequipment:combines,48;graintrucks,16;shredders,22;balers,36;stackers,6.bStartofharvestisSeptember15.cLargerectangularbalerstover can be easily compacted into pellets for easy transport and storage [21,22]. Economics and energy input to the pelleting operation were obtained from Mani et al. [23].
3.3. Stover transport
Stover in the form of bales, chops and pellets are usually transported by trucks. Bales are transported using flatbed trucks. The ground (chopped) and pelletized biomass is transported using truck boxes. In our analysis, the biomass is loaded onto trucks and transported to an integrated combined heat and power (CHP) and ethanol plant daily throughout the year. For bale transport, bales are stacked on a flatbed trailer to the maximum height of 4 m (above ground). Roughly 36 rectangular bales (1.2 1.2 2.4 m) are placed on a 14.6 m long flat bed. Larger trailers with more axles are available when allowed by local transport regulations. For bale loading and unloading, loaders equipped with bale grabbers were used. The bale grabbers remove one or two bales from the stack at a time and place them on the deck of the trailer. A full bale load weighs roughly 18 Mg assuming 0.5 Mg per bale on dry basis (0% moisture content). The truck box is 2.4 m wide 2.4 m high 12.6 m long with a capacity of roughly 70 m3. Tests with grinders have shown that grinding biomass to a size less than 5 mm can result in a bulk density of about 160 kg m 3[21]. This will yield a load of roughly 11 Mg per truck box. The pellets can be Table 3 Cost and energy analyses of biomass pelleting process [23]. Processes Cost ($ Mg 1) Energy (GJ Mg 1) Bale tub grindinga 5.72 0.15 Hammer mill 0.95 0.59 Pellet mill 3.31 0.31 Pellet cooler 0.34 0.46 Screens 0.16 0.07 Miscellaneous equipment 2.77 0.08 Labor 12.74 0.18 Land and building use 0.26 Sub-total 26.25 1.84 Total (plus 15% profit) 30.19 a Data obtained from Wright et al. [19]. loaded onto truck boxes and transported to the ethanol plant. We assume a bulk density of 560 kg m 3 for pelletized or cubed stover. A 70 m3 truck box can hold roughly 40 Mg. This is an efficient way of moving biomass assuming that carrying heavy loads is permitted on the local roads. #p#分页标题#e#
3.4. On-site storage and fuel preparation
For storage, a covered building with a flat floor for bales and chops is considered. For pellets, we specify steel bins with a flat floor. The area footprint for three forms of biomass was 2780 m2 for bales, 3870 m2 for ground stover and 348 m2 for pelletized biomass. The difference in areas was due to bulk density and the area allowed for movement of handling equipment. The storage building costs were estimated at 107$ m 2, 161$ m 2 and 215$ m 2, for storing bales, ground (chops) and pellets. The storage building for chopped biomass was more than that for bales because retaining walls are needed to hold the pile of biomass. The most expensive storage is steel bins with filling and emptying auxiliaries and aeration equipment. Solid fuels require on-site preparation to be used in the boiler. The combustion system for this project will accommodate pulverized biomass/solid fuels. Corn stover delivered to the ethanol plant will be in one of three forms: square bales, ground chops, or pellets. The aforementioned three forms of biomass can be easily handled in the existing grain handling facilities with necessary modifications or additional processing/handling equipment. Ground biomass and square bales may be stored under cover or in the open sheds. Each of the biomass formats (Fig. 2) requires different pre-treatment before it can be fed to the burner. Square bales require destringing, de-baling (primary shredding), fine grinding (hammer milling) and are then conveyed by a pneumatic
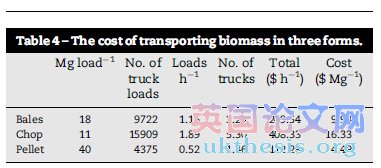
Table 4 The cost of transporting biomass in three forms. Mg load 1 No. of Loads No. of Total Cost truck h 1 trucks ($ h 1) ($Mg 1) loads Bales 18 9722 1.16 3.24 249.54 9.98 Chop 11 15909 1.89 5.30 408.33 16.33 Pellet 40 4375 0.52 1.46 112.29 4.49
biomass and bioenergy 34 (2010) 75–81 Operations
Table 5 Estimated cost of receiving, storing, and preparing biomass for boiler use. Baled stover Chopped stover Pelletized stover Explanation Cost ($ Mg 1) Explanation Cost ($ Mg 1) Explanation Cost ($ Mg 1) Receiving Storing Reclaiming biomass Fine grinding Unload and stack bales Enclosed, free standing, stacked bales Stacker, belt conveyor, de-stringer, de-baler Hammer mill 2.22 4.77 4.34 Front-end loader and piler Enclosed reinforced bearing walls for piled stover Front-end loader, conveyor 1.10 6.62 1.40 Dump in pit, elevatea Steel bin with overhead distribution Bin unloader Hammer mill Pneumatic, airlock, 0.00 1.27 1.40 5.41 2.21 (t30% overhead) 5.41 Hammer mill 5.41 Delivery Pneumatic airlock, blower 2.21 Pneumatic, airlock, blower 2.21 Total 24.64 21.76 blower a Use the existing grain pit and elevator. 13.38 feeding system to the boiler. To manage dust during fine grinding, the ground biomass will be collected in the cyclone system. Dry chops and pelletized biomass require only a single stage grinding. Ground biomass may not require regrinding. A similar type of fuel preparation system is currently used in the Chariton Valley power generation system [24,25]. #p#分页标题#e#
4. Results and discussions 结果与讨论
4.1. Cost of biomass collection
Table 2 summarizes IBSAL’s output for collection operations including size, production rate, and number of equipment to make it possible to complete each operation at a specific date. Collection operation requires 20 shredders, 36 balers, and six bale stackers to complete the collection operations for an ethanol plant. The output includes completion dates for each operation. For corn stover, the net amount collected was 1.399Tg at 38$ Mg 1 delivered cost using the IBSAL model. The cost includes $10 payment to the producer and 15% of total collection costs as profit assuming the work is done by a custom operator.
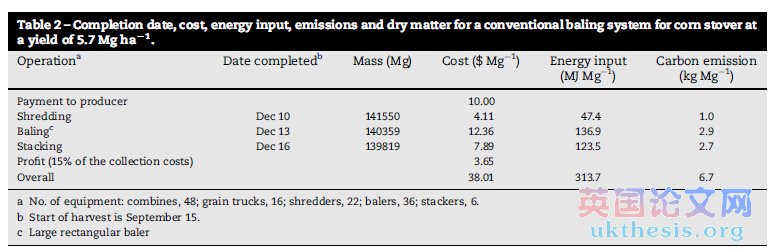
Table 2 lists the amount of energy input to
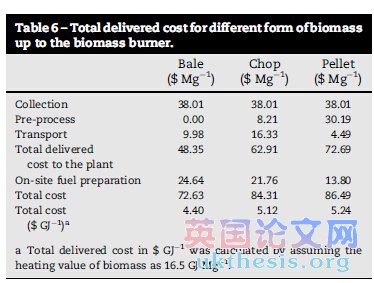
Table 6 Total delivered cost for different form of biomass up to the biomass burner. Bale Chop Pellet ($ Mg 1) ($ Mg 1) ($ Mg 1) Collection 38.01 38.01 38.01 Pre-process 0.00 8.21 30.19 Transport 9.98 16.33 4.49 Total delivered 48.35 62.91 72.69 cost to the plant On-site fuel preparation 24.64 21.76 13.80 Total cost 72.63 84.31 86.49 Total cost 4.40 5.12 5.24 ($ GJ 1)a aTotaldeliveredcostin$GJ 1wascalculatedbyassumingtheheatingvalueofbiomassas16.5GJMg 1.the power equipment due to diesel fuel consumption. All the mass units are reported throughout on a dry basis (0% moisture content). The input energy required to collect corn stover is 313.7 MJ Mg 1. To put this energy use in perspective, the amount of energy in a dry Mg of stover is roughly 17 GJ. The amount of energy used to power equipment is roughly 2% of the energy content of biomass. Table 2 also lists the amount of carbon emission as a result of burning diesel fuels in the collection machinery.
4.2. Pre-processing cost
The costs for chopping involve a loader with an operator (1.42 $ Mg 1), and an industrial grinder (5.72 $ Mg 1), for a total of 7.14$ Mg 1. We increased the cost by 15% to account for profit (return on investment) to 8.21 $ Mg 1.
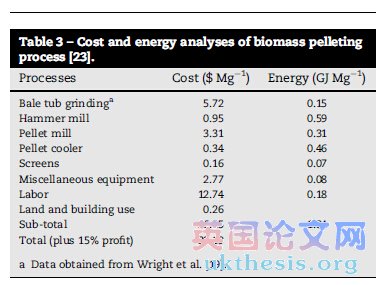
Table 3 shows the breakdown of cost and energy demand for the biomass pelleting process. The pelleting plant has a production capacity of 6Mg h 1 with annual production of 45 Gg [23]. Roughly three pellet mills are needed to process the entire biomass for the ethanol plant. The pellet plant operates 24 h for 310 days annually (annual utilization period 85%). The dry biomass is used as a feedstock for the pellet plant and that eliminates the drying operation. Total cost of pelleting was about 30.19 $ Mg 1 of pellets with an energy requirement of 1.84 GJ Mg 1. About 11% of the biomass energy is used to produce pellets. Cost of pelleting biomass can be further reduced if a larger capacity pellet plant (12–15 Mg h 1) is considered [23]. #p#分页标题#e#
4.3. Transport cost
Transport cost of biomass is one of the major cost components in the entire supply system. Biomass transport cost varies with form of biomass and distances to be transported. Table 4 summarizes calculations for the cost of transporting biomass. The table lists Mg per load, total number of truck loads to transport 140 Gg year 1 and the number of required loads per hour. Then, the number of truckloads required for a given average speed of 50 km h 1 and 140 km distance traveled (70 km each way) is calculated. The total $ h 1 is the product of the number of trucks required by the cost of one
biomass and bioenergy 34 (2010) 75–81 truck load (at 77 $ h 1). Total $ h 1 divided by 25 Mg h 1 gives the $ Mg 1 that is $10 for transporting bales, $16 for transporting ground biomass and $4.5 for transporting pellets.
4.4. On-site fuel storage and preparation
Table 5 itemizes the costs of receiving, storing, reclaiming and final fine grinding of biomass for immediate delivery to the boiler room. Bales are received at the ethanol plant and will be stacked under a roof. Chopped (shredded) biomass is received in silage type truck boxes. The load is dumped on a concrete floor and piled using a front-end loader. The existing grain pit and grain elevator legs at the ethanol plant can serve to unload and distribute pellets to the bin. We assumed 1.5 Gg storage of biomass will be adequate for 3 days of operation at 500 Mg day 1. The cost of reclaiming bales is higher than those for chops and pellets because each bale has to be placed individually on a conveyor belt. The strings are cut and removed automatically as the bales advance towards a primary shredder (debaler). These processes are not necessary for the shredded or pelletized feedstock. The shredded feedstock or pellets are ground into fine particles in a hammer mill. Table 5 also estimates the cost of pneumatic conveying of ground biomass to the burner at 2 $ Mg 1. An overhead of 30% is added to the costs for contingency, and any extra labor requirement.
4.5. The total cost of biomass fuel delivered to the burner
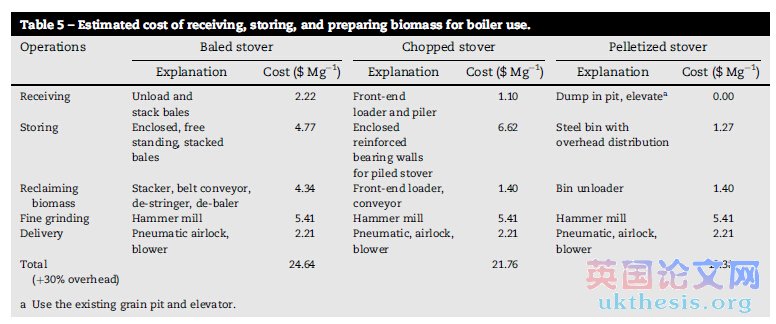
Table 6 summarizes the total delivered cost of different biomass formats to the burner. Pelleted biomass has the highest delivery cost of 86 $ Mg 1 mostly because of the high cost of making pellets from bales. Next is the delivered cost of chopped biomass at 84 $ Mg 1. The square bale format has the lowest delivered cost of 73 $ Mg 1. The delivered cost of switchgrass in the form of bales was about 81 $ Mg 1 for 10Mg ha 1 biomass yield [26]. Biomass can be supplied to produce heat and power to the ethanol plant at a price lower than the cost of DDG (100 $ Mg 1) currently sold in the market [4]. A cost difference of 10–20 $ Mg 1 of feedstock would make a considerable difference in the annual energy saving for the ethanol plant. Overall, for the scenarios and the distance of 70 km transport, pellets are the most expensive form of biomass delivered to the burner, followed by chops and square bales. For corn stover, opportunity still exists for reducing the delivered cost of stover through single pass harvesting, compressed bulk transport and granulation of biomass. 5. Concluding remarks A systematic cost analysis of corn stover collection, pre-processing and transport operations combined estimated as 48, 63 and 73$ Mg 1 for baled, chopped and pelleted biomass respectively. An additional 25 $ Mg 1 is required to prepare baled biomass to make it suitable for a combustion process. Pelleted biomass has the least fuel preparation cost of 14 $ Mg 1, due to its low on-site storage cost. Collection cost of biomass (38 $ Mg 1) was the largest cost factor in the entire supply system, which can be further reduced if a whole crop harvesting system is developed and used. Research work has already been initiated to estimate the stover collection cost using a whole crop harvesting system. Transport cost of chopped biomass was the highest due to low bulk density. Compaction of chopped biomass in the truck to increase bulk density may reduce the transport cost further by increasing the payload per truck. Although the transport cost of pelleted biomass is less, the pellet production cost is a technical barrier. Granulation of biomass to increase bulk density may reduce the cost of pre-processing. Granulation is a process of increasing the size and bulk density of biomass particles using limited shear forces and liquid binders. Biomass granulation will also reduce the on-site fuel preparation cost, as granules do not require fine grinding and an expensive pneumatic handling system. Apart from the high cost of compacting biomass into pellets (30 $ Mg 1), pellets can be easily stored for long periods, similar to grains, and become an attractive form of fuel for domestic and central heating applications. Further reduction in biomass delivered cost is possible if more efficient collection and transport systems are developed. We envision that creating demand for biomass will build infrastructure for biomass fuels, which may further reduce the cost of biomass. Successful use of biomass in Europe is mainly because of well established infrastructures to collect, transport and store biomass fuels. For example in Holland, Denmark and Sweden, the use of waterways to transport bulk biomass has considerably reduced the cost of biomass transport. #p#分页标题#e#
Acknowledgement 致谢
The authors acknowledge Oak Ridge National Laboratory (ORNL) and Office of Biomass Program, US Department of Energy (DOE), for providing funding to conduct this research.
references 文献
[1] American Coalition for Ethanol (ACE). U.S. Ethanol Production. (accessed June, 2006). [2] Carbon Sequestration Leadership Forum (CSLF). An energy summary of the United States of America. [3] Clayton M. Carbon cloud over a green fuel. 2006; (accessed in July, 2006). [4] Morey RV, Tiffany DG, Hatfield DL. Biomass for electricity and process heat at ethanol plants. ASABE paper # 056131. St. Joseph, MI: ASABE; 2005. [5] Wang M, Saricks C, Santini D. Effects of fuel ethanol use on fuel-cycle energy and greenhouse gas emissions. Argonne National Laboratory, ANL/ESD-38; 1999. [6] Shapouri H, Duffield JA, Wang M. The energy balance of corn ethanol: an update. Agricultural economic report No. 813. Washington, DC: United States Department of Agriculture (USDA); 2002.
biomass and bioenergy 34 (2010) 75–81 [7] Shapouri H, Duffield JA, Wang M. The energy balance of corn ethanol revisited. Transactions of the ASAE 1999;46(4): 959–68. [8] Sokhansanj S, Turhollow AF, Wilkerson EG. Development of the Integrated Biomass Supply Analysis and Logistics Model (IBSAL). Technical Memorandum ORNL/TM-2006/57. Oak Ridge, TN: Oak Ridge National Laboratory. [9] EXTENDSIM. Users manual. Version 7.0.5. San Jose, CA: Imagine That Inc; 2008. [10] Sokhansanj S, Kumar A, Turhollow AF. Development and implementation of Integrated Biomass Supply Analysis and Logistics Model (IBSAL). Biomass & Bioenergy 2006;30:538–47. [11] Sokhansanj S, Mani S. Modeling of biomass supply logistics. In: Bridgewater AV, Bobcock BDG, editors. Science in Thermal and Chemical Biomass Conversion, Vol. 1. Newbury Perks, UK: CPL Press; 2006. p. 387–403. [12] ASABE, 2007. Standards. ASAE D497.5 FEB2006, Agricultural Machinery Management Data, 362_369. St. Joseph, MI: ASABE. [13] Sokhansanj S, Turhollow AF. Baseline cost for corn stover collection. Applied Engineering in Agriculture 2002;18(5):38–43. [14] Cundiff JS, Marsh LS. Harvest and storage costs for bales of switchgrass in the southern United States. Bioresource Technology 1996;56:95–101. [15] Cundiff JS. Delayed harvest of switchgrass. 1994–1995 Annual Report. Biological Systems Engineering Department. Blacksburg, VA; 24061-0303: Virginia Tech; 1995, 16 p. [16] Cundiff JS. Simulation of five large round bale harvesting systems for biomass. Bioresource Technology 1996;56:77–82. [17] Sokhansanj S, Turhollow AF, Cushman J, Cundiff J. Engineering aspects of collecting corn stover for bioenergy. Biomass and Bioenergy 2002;23:347–55. [18] Stinger Inc. 2006. Stinger Model 6500[19] Wright CT, Pyrofogle PA, Stevens NA, Hess JA, Radtke CW. Value of distributed preprocessing of biomass feedstock to a bioenergy industry. ASABE paper no. 066151. St. Joseph, MI: ASABE; 2006. [20] Sokhansanj S, Turhollow AF. Biomass densification – cubing operations and costs. Applied Engineering in Agriculture 2004;20(4):495–9. [21] Mani S. A systems analysis of biomass densification process. PhD dissertation, Department of Chemical & Biological Engineering. Vancouver, BC: University of British Columbia; 2005. [22] Mani S, Tabil LG, Sokhansanj S. Effects of compressive force, particle size and moisture content on mechanical properties of biomass pellets from grasses. Biomass and Bioenergy 2006;30:648–54. [23] Mani S, Sokhansanj S, Bi X, Turhollow AF. Economics of producing fuel pellets from biomass. Applied Engineering in Agriculture 2006;22(3):421–6. [24] CV-RC&D. Chariton Valley Biomass Project Design Package. Prepared for USDOE under contract #DE-FC36-96 G010148. Centerville, IA: Chariton Valley RC&D; 2002. [25] Chariton Valley Biomass Projects (CVBP). Chariton valley biomass projects – Department of energy project update; 2004. (accessed March, 2006). [26] Sokhansanj S, Mani S, Turhollow AF, Kumar A, Bransby D, Lynd L, et al. Large scale production, harvest and logistics of switchgrass – current technology and envisioning a mature technology. Biofuels, Bioproducts & Biorefining 2009;3: 124–41. #p#分页标题#e#