abstract 摘要
在过去的十年里,英国政府的政策支持可再生能源(RE)已经在英国的能源快速增长的核心而不是真正的市场力量。政策支持已经通过本文中讨论的各种工具,特别注意给定的共同发射和运输燃料。英国能源部门仍占主导地位的大型化石燃料发电厂,这不利于引进的重新。而一个例外的是共烧在2005产生2.5太瓦时,另一个相当大的潜在利益是使用生物燃料(生物柴油和生物乙醇)运输,虽然目前的贡献仍然很小(2005的燃料总销售的0.18%)。在2005,英国使用了140万吨生物质的共烧,主要是进口的,而存在相当大的潜力,进一步扩大应政策激励继续。它也被普遍接受,英国将不得不依赖于进口,如果它是履行其5%个最低的生物燃料的2010和2015,在10%同意。两部门目前的大好机遇国际能源贸易,尽管国内的生产潜力,如在本文中证明。此外,碳和可持续性报告即将出台,应该鼓励更大的报告沿着供应链,鼓励最可持续的生物燃料贸易。
In the past decade or so, the British government policy support for renewable energy (RE) has been at the core of the rapid growth of bioenergy in the UK rather than genuine market forces. Policy support has been through various instruments which are discussed in this paper, with particular attention given to co-firing and transport fuels. The UK energy sector remains dominated by large fossil fuels power plants and this does not facilitate the introduction of RE. One exception is co-firing which in 2005 generated 2.5 TWh. Another area of considerable potential and interest is the use of biofuels (biodiesel and bioethanol) for transport, although current contribution remains still very small (0.18% of total fuel sales in 2005). In 2005, the UK used 1.4 million tonnes of biomass in co-firing, mostly imported, while considerable potential exists for further expansion should policy incentives continue. It is also generally accepted that the UK will have to rely largely on imports if it is to fulfil its 5% minimum biofuel blends agreed for 2010 and 10% in 2015. Both sectors present excellent opportunities for international bioenergy trade, despite the domestic production potential, as demonstrated in this paper. In addition, the forthcoming introduction of carbon and sustainability reporting should incentivise greater reporting along the supply chain, encouraging trade in the most sustainable biofuels.
1. Introduction 介绍
In recent years, the UK bioenergy market has grown considerably, consuming over 33 PJ of biomass-derived energy in 2006 (see Table 3). This figure is likely to grow as the obligation to produce renewable electricity becomes stricter and the obligation to use biofuel blends in transport takes effect. The purpose of this paper is to provide an overview of how international bioenergy trade has developed in the UK and what role imported biomass is likely to play in the energy mix up to 2010. The paper also discusses critical issues in determining the greenhouse gas (GHG) emissions mitigated Corresponding author. Tel.: +44 20 7594 9313. E-mail address:
[email protected] (M. Perry). 0961-9534/$ -see front matter & 2008 Elsevier Ltd. All rights reserved. doi:10.1016/j.biombioe.2008.01.004 through bioenergy and the development of a mandatory carbon reporting framework for UK biofuels. The paper focuses on the technological, institutional and policy context that has driven UK bioenergy trade to its current level and is likely to continue in the near future. Section 2 provides an overview of the UK energy sector, demonstrating that bioenergy, excluding refuse-derived forms such as landfill gas combustion, has traditionally played a minor role in the UK energy system. Section 3 examines individual UK bioenergy markets, and their policy drivers, in greater detail. It pays particular attention to the role of co-firing biomass in coal-fired power stations and the introduction of low-blend
BIOMASS AND BIOENERGY 32 (2008) 688– 701 Electricity Generated from Renewable Sources: 2000 - 2006 Electricity - GWh 20,000 18,000 16,000 14,000 12,000 10,000 8,000 6,000 4,000 2,000 -2000 2001 2002 2003 2004 2005 2006 Year Other (mainly wind and hydro) Landfill gas Sewage sludge digestion Municipal solid waste combustion Co-firing with fossil fuels Fig. 1 – Electricity generated from renewable sources, 1998–2005. Source: [1]. Notes: (1) Waste material includes only biodegradable element, (2) other includes straw, short rotation coppice, farm waste digestion and poultry litter, meat and bone combustion. biofuels. These sources account for a large share of UK bioenergy use, have potential for significant further growth within the existing infrastructure and have used mainly imported feedstocks. One result of the UK’s traditionally neutral approach to bioenergy is a lack of integrated analysis that focuses on bioenergy supply and demand. Section 4 attempts to rectify this by combining elements of existing assessments into an indicative estimate of both the UK demand for bioenergy and the potential domestic bioenergy resource in 2010. Section 5 discusses some of the issues that are important in determining the GHG emissions savings that can be achieved through importing biomass and how these issues could be addressed through the UK’s carbon reporting framework for biofuels. Findings are discussed in Section 6 and conclusions drawn in Section 7.
2. Overview of UK energy sector 英国能源部门概述
Over the last 30 years, the UK has switched from being a net exporter, becoming a net importer of primary energy in 2004, when it imported about 5% of consumption. Production of North Sea oil and gas peaked at levels of 3.6 and 4.5 EJ in 1999 and 2000, respectively [1]. The period from 1970 to 2005 also saw a shift from coal to gas as the main source of primary energy, and towards greater use of gas and electricity in final consumption. Natural gas accounted for 41% of primary fuel consumption for energy use in 2004 compared to 5.4% in 1970. The use of coal as a primary fuel also fell from 47.1% of total use to 16.7%. The distribution of both electricity and gas in the UK follows a highly centralised model. The national gas grid is a comprehensive network that supplies around three quarters of heat consumed [2]. In the electricity market, output is traded around the UK. Most notably, electricity is produced in Scotland and Yorkshire and transmitted to the South of England where demand is highest [3]. The centralised nature of the UK energy system is reinforced by the planning regime which has been identified by the Government as a barrier to the deployment of new energy projects [4,5]. The increase in the use of gas as a primary fuel is partly due to its increased use in electricity generation over the 1990s, the so-called dash for gas, rising from 1% of natural gas consumption in 1990 to 30% in 2004. However, the use of gas in electricity generation fell year-on-year in 2005 and 2006. The UK electricity sector is dominated by coal, gas and nuclear sources. In 2006, 36% of electricity supplied came from gas, 38% from coal and 18% from nuclear and 4.6% of electricity generation came from renewable sources [1]. The total use of renewable heat and power sources in 2005 was four times the 1990 level. Renewable heat use has fallen due to tightening of regulations for burning wood waste (see Section 3.2) [1]. Despite this, wood is the largest feedstock for renewable heat in the UK, accounting for 81% of renewable heat (both commercial and domestic) in 1996 and 70% in 2004. In 2006, biomass from sources other than sewage and refuse accounted for 3.3 TWh (11.9 PJ) or 18.3% of renewable electricity generation. The largest generators were hydro (25.3%), landfill gas (24.3%) and wind (23.3%). In Fig. 1, biofuels are represented by the shaded bars. Although most of this biomass is waste-derived, co-firing of biomass with coal has also become a major source of renewable electricity, accounting for 13.9% of total renewable electricity generation in 2006. #p#分页标题#e#
3. UK bioenergy markets 英国生物能源市场
This section examines the bioelectricity, biomass heat, co-firing and transport biofuel markets. It also assesses the impact on bioenergy of different policies that have incentivised renewable energy (RE) in recent years.
Table 1 shows the quantities of biomass used in the generation of heat and
BIOMASS AND BIOENERGY 32 (2008) 688– 701 Table 1 Biomass combustion for heat and electricity, UK 2006 Energy product Fuel Heat/ input—gross electricity calorific value generated (PJ) (PJ) Heat from biomass 19 – Of which landfill gas, 4 – sewage sludge and organic MSW Electricity from biomass 98.4 24.5 Of which landfill gas, 87.2 21.5 sewage sludge and organic MSW CHP—Heata 4.6 3.2 CHP—Electricitya 6.2 2.2 Co-firing with fossil fuels 34.7 9.1 Source: [66]. a CHP data are a subset of the totals for heat and electricity from biomass. electricity in 2006. Biomass-based heat and electricity is dominated by the use of landfill gas and refuse-derived fuels. The exception to this is co-firing with coal, examined in Section 3.2.
3.1. Renewable energy policy and the development of
bioenergy From 1990 to 2002, the Non-Fossil Fuel Obligation (NFFO) was the main Government support measure for RE. NFFO consisted of a Fossil Fuel Levy charged to electricity suppliers and a series of feed-in tariffs, funded by the levy, that supported renewable electricity. NFFO contracts were awarded in a series of tranches, the last of which was awarded in 1998, with the final contract expiring in 2018 [6]. From 1990 to 1999, biomass accounted for 221 MWe of the 3600 MWe awarded in NFFO contracts. In 2005, ongoing biomass projects accounted for 81 MWe1 —around 7% of NFFO capacity [1]. In 2002, NFFO was replaced by the Renewables Obligation (RO). This is a system of tradable permits (known as ROCs—Renewables Obligation Certificates). One ROC is awarded for each MWh of electricity generated from eligible renewable sources. Licensed suppliers of electricity are obliged to supply a given percentage of their electricity from eligible renewable sources. They can fulfil this obligation by generating ROC-eligible electricity themselves, buying ROCs from other generators or paying a buyout price. At the end of the year, the buyout fund—the total value of the buyouts purchased—is redistributed to ROC holders, providing a further incentive to produce ROCs. The initial level of RO was 3%, rising to 15.4% in 2015 with a recent promise by the Government that it will rise to 20% ‘‘when justified by growth in renewable generation’’ [5]. The RO is guaranteed to remain in place until at least 2027, in order to assure investors that a long-term market exists for renewable electricity. Other policies that favour bioenergy relative to fossil equivalents are the Climate Change Levy (CCL) and European Emissions Trading Scheme (EU-ETS). CCL is a surcharge levied on the use of fossil fuels and electricity in the commercial, industrial and public sectors [7]. The level of the charge ranges from 0.15 pence (hc0.222) per kWh for natural gas to 1.17 pence (hc1.68) per kg for solid fuels, while biomass is exempt. EU-ETS is a cap-and-trade scheme in which large industrial plants must produce emissions permits for each tonne of CO2 they emit, but where biomass is counted as carbon-neutral. The ability of EU-ETS to encourage the take up of carbon abatement systems such as bioenergy depends on the carbon price, which in turn depends on the total level of carbon abatement demanded at European level. Since inception, the carbon price has been volatile, leading to uncertainty over how the abatement incentive it offers will develop. EU-ETS allowances began trading at under 10 h/tCO2 in January 2005, rose to over 30 h/tCO2 by April 2006 but then fell to below 10 h/tCO2 once more [8]. There are several key differences between the support offered to biomass through NFFO and the RO. Most importantly, NFFO is a competitive tendering process awarding support only to specific projects. Biomass contracts were awarded in the first and third tranche [9]. Contracts were awarded in the fourth tranche but exclusively to advanced combustion projects such as pyrolysis and gasification. A competitive tendering process has the advantage of being able to award contracts only to the lowest cost projects. However, competition among bidders reduces the value of the eventual tariff to the generator. Only a fraction of NFFO contracts resulted in the eventual commissioning of a biomass facility. This was partly due to planning difficulties and partly because some developers could essentially treat NFFO contracts as an option to sell bioelectricity rather than a firm commitment to do so [10]. In 2003, seven NFFO biomass contracts (105 MWe) were active from a total awarded of 22 [9] while none of the advanced combustion contracts awarded have yet been commissioned and have gone into liquidation [9,11]. The support offered by the RO is not project-specific. One ROC is awarded for every MWh of renewable electricity generated. This makes the RO ‘‘technology neutral’’ as wind, wave, small-scale hydro, solar and biomass are supported equally, automatically favouring projects and technologies deemed most cost effective in the private sector. Under the RO, generators are the sellers of ROCs while suppliers buy them in order to fulfil their obligation. The ROC market is limited in size by the suppliers’ obligations and the level of the buyout price meaning that any generator considering investing in renewable electricity must consider the possibility of a fall in the ROC price resulting from oversupply. 1 In this figure, biomass includes energy crops and agricultural and forestry wastes. Municipal and industrial waste, landfill gas, 2 All currency conversions performed on 30/09/2007, as per sewage gas and waste to energy are excluded. #p#分页标题#e#
BIOMASS AND BIOENERGY 32 (2008) 688– 701 In the 2004–2005 compliance period, 10 million ROCs (from a total of 13 million) were issued to generation from wind, landfill gas or co-firing. Co-firing accounted for 25% of total ROCs issued. The percentage of co-fired ROCs that each supplier can include in their ROC claim was also capped at this level to limit the dominance of co-firing within the RO [12]. In April 2006 this limit fell to 10%. As a result of the cap there are effectively two separate ROC markets, one for co-fired ROCs, that suppliers can only use in limited proportions and one for all other ROCs. In July 2007, standard ROCs sold for an average auction price of h69 (£48.12) while co-fired ROCs sold for h65 (£45.10) [13]. The ROC status of co-firing has also undergone frequent change since the inception of the RO. ROC eligibility for co-firing was originally due to the end in 2011 with an obligatory 75% of feedstocks coming from energy crops from 2006 onwards. In 2004, the regulations were relaxed to require energy crops from 2009 with ROC eligibility expiring in 2016. Since co-firing requires relatively little capital investment compared to other forms of renewable electricity, the RO regulations explicitly rule out grandfathering of co-firing support, under which co-firing investments would be protected from adverse conditions created by any subsequent regulatory changes. Additional uncertainty exists over the precise operational definitions of terms in the RO legislation (e.g. precisely what can be eligible as an energy crop) and whether the burning of a given feedstock will be permitted by the local Environment Agency [14]. These factors add uncertainty to the decision making of operators considering investing in co-firing projects, including the need to reach a conclusion as to what future policy constraints may eventually emerge. Two important points can be derived from the above information. Firstly, the level of biomass co-firing is constrained by an arbitrary cap rather than by technical or economic potential. Secondly, current ROC prices probably provide more support than is necessary for co-firing to be economically viable, though the ROC framework does not provide long-term certainty to decision makers. These factors make co-firing a profitable activity at the margin but do not encourage long-term investment, an issue noted in Ernst & Young [15] where co-firing investments are assumed to require a shorter payback period. In these circumstances, a removal of the cap on co-firing would substantially increase the levels of biomass co-fired but this would be at the expense of other ROC-eligible projects. In order to increase the levels of co-firing without jeopardising other renewables, the Government is proposing to alter the RO from 2007 [16]. Under this proposal, the RO would be ‘‘banded’’. Co-fired electricity would receive less than 1 ROC per MWh while renewables that are not currently viable but have long-term potential, such as dedicated bioelectricity plants and offshore wind, would receive more than 1 ROC per MWh. #p#分页标题#e#
3.2. Dedicated bioelectricity plants
As of August 2007, there were 19 dedicated biomass plants in the UK with an installed generating capacity of 50 kW or more, a combined generating capacity of 226 MWe [17]. Several of these plants were designed to burn-specific animal or plant by-products and many generate income from the production of by-products such as fertiliser or from the disposal of agricultural waste. Details of the largest plants (capacity X5000 kW) are given in Table 2. The two largest bioelectricity plants, Wilton 103 and Stevens Croft,4 were both commissioned in 2007 and are designed for combustion of short rotation coppice (SRC) and other woodfuel. Hypothetically, dedicated bioelectricity plants could use imported feedstock rather than rely on local biomass resources. Such a policy would entail additional risks relating to security of feedstock supply, particularly since electricity producers face competition from other buyers for feedstocks. Recently, two projects have investigated the possibility of running power stations using imported vegetable oil (primarily palm oil). One project, a district heating and CHP scheme in Southampton is still in the planning stage.5 The other project, involving the conversion of an oil-fired power station to burning 100% biomass, did not take place. The reason given by the operator was the difficulty in sourcing large volumes of sustainably sourced palm oil [18]. In the Government report Economic Analysis of Biomass Energy [19], analysis of dedicated bioelectricity plants does not include the possibility of using imported feedstocks. The prices quoted for domestic biomass (£1.4 (h2)–£3.9 (h5.6)/GJ) compare favourably to prices of imported biomass (£3.5 (h5)–£5.5 (h7.9)/GJ). However, since utilisation of domestic biomass sources such as straw, SRC and woodfuel (including contaminated wood waste) may require the establishment of dedicated supply chains and processing equipment whereas some imported materials such as palm kernel expeller (PKE), olive cake and wood pellet can be purchased in large quantities and require less processing for combustion, the use of imported biomass as part of the feedstock portfolio in dedicated UK bioelectricity plants should not be ruled out.
3.3. Co-firing
Co-firing of biomass in coal-fired power stations began on a commercial scale with the establishment of the RO in 2002. In 2005, co-firing generated 2.5 TWh of electricity, a 148% increase on the 2004 level. This made co-firing the third largest generator of renewable electricity behind large-scale hydro and landfill gas and ahead of wind [1]. The technical potential for co-firing depends on the scale of coal-fired electricity production and the extent to which plant operators are willing to make modifications to increase the proportion of biomass co-fired. There are 14 coal-fired power stations with a stated capacity of over 1 GWe in the UK.6 The total capacity of the UK’s coal-fired power stations is 29 GWe. This includes facilities with a combined capacity of over 7 GWe that have chosen to close by 2016 rather than fit the emissions control equipment to comply with the European Large Combustion Plant Directive [20]. The levels of co-firing that can be achieved in a given plant depend on the types of biomass and the co-firing techniques 3 subcritical pulverised fuel plants. #p#分页标题#e#
BIOMASS AND BIOENERGY 32 (2008) 688– 701
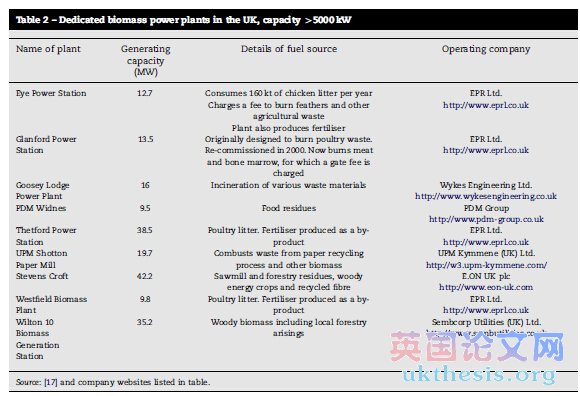
Table 2 Dedicated biomass power plants in the UK, capacity 45000 kW Name of plant Generating Details of fuel source Operating company capacity (MW) Eye Power Station 12.7 Glanford Power 13.5 Station Goosey Lodge 16 Power Plant PDM Widnes 9.5 Thetford Power 38.5 Station UPM Shotton 19.7 Paper Mill Stevens Croft 42.2 Westfield Biomass 9.8 Plant Wilton 10 35.2 Biomass Generation Station Consumes 160 kt of chicken litter per year Charges a fee to burn feathers and other agricultural waste Plant also produces fertiliser Originally designed to burn poultry waste. Re-commissioned in 2000. Now burns meat and bone marrow, for which a gate fee is charged Incineration of various waste materials Food residues Poultry litter. Fertiliser produced as a byproduct Combusts waste from paper recycling process and other biomass Sawmill and forestry residues, woody energy crops and recycled fibre Poultry litter. Fertiliser produced as a byproduct Woody biomass including local forestry arisings EPR Ltd. [17] and company websites listed in table. used. Tests undertaken at facilities designed to emulate the performance of UK plants have demonstrated that up to 20% biomass, by thermal input, can successfully be co-fired7 [21]. The main operational issues encountered during the tests were the build-up of heavy, pernicious deposits when co-firing miscanthus, corrosion of superheater and boiler wall material when co-firing cereal co-product at 20% thermal input and difficulties in finding effective storage and feeding mechanisms for the biomass. The trials also concluded that the input of 10% biomass by thermal input did not significantly affect ash deposition behaviour or increase corrosion rates, with the possible exception of cereal co-product. Techniques available for co-firing biomass with fossil fuels include: Co-milling—it is estimated that the coal mills used at UK plants can mill a maximum of 10–15% biomass by mass [12,21]. A wide variety of feedstocks can be co-milled in small quantities. However, it is difficult to co-mill large quantities of material that is moist and fibrous, such as wood chip [22]. Direct injection—several plants have invested in direct injection mechanisms, allowing biomass to enter the main boiler directly [23]. They offer greater flexibility in feed-stocks since the biomass bypasses the coal mill. A simple 7 16% SRC, miscanthus and PKE and 20% wood, straw and cereal co-product trialled. direct injection mechanism requires relatively little capital investment and can reportedly repay the necessary investment in 6 months [14]. Advanced co-firing techniques include gasification or pyrolysis of biomass prior to combustion. Biomass and fossil fuels can also be co-fired indirectly, for example in facilities where separate boilers are connected to a common steam turbine. A more detailed overview of advanced co-firing techniques is available in Perry and Rosillo-Calle [14]. In 2006, 35 PJ of biomass was co-fired and 1502 PJ of coal used in electricity generation [1], giving a nationwide co-firing rate of 2.26% by energy input. Assuming that an overall co-firing rate of 10% is feasible, existing plant could co-fire over four times the amount used in 2006. Without accounting for efficiency losses that may occur as more biomass is co-fired,8 this would be sufficient to produce 39.8 PJ of electricity from 155 PJ of biomass. However, as higher proportions of biomass are co-fired, greater plant modifications, such as direct injection mechanisms, are likely to be needed and problems of deposition, corrosion and fuel storage and handling could become more significant. 8 According to Tillman, co-firing 10% biomass, by mass, is likely to lead to a loss in boiler efficiency of 0.5% compared to a 100% coal [24]. #p#分页标题#e#
BIOMASS AND BIOENERGY 32 (2008) 688– 701 3.4. Renewable heat In 2006, 20.5 PJ of renewable sources were used to generate heat, of which 92% came from biomass. In 1996 this figure stood at 37 PJ. In the past 10 years the number of industrial sites combusting waste wood has fallen sharply while there has been little change in the combustion of straw, municipal solid waste (MSW) and domestic woodfuel [1]. The decline in combustion of industrial wood waste has been attributed to more stringent emissions controls [1]. In particular wood that has, at some point, been treated with halogenated organic compounds or heavy metals is usually classed as a waste and therefore can only be combusted in plants that comply with the emissions limit values stipulated in the 2002 Waste Incineration Regulations [12,25].9 In the current policy framework, renewable electricity is systematically favoured over heat as a market for biomass since the RO rewards renewable electricity generation while no such policy exists for heat. This makes it relatively more attractive to use biomass in the generation of electricity [26]. Some Government funds have been made available in recent years to provide demand-side support for renewable heat. This support comes in the form of grants for community organisations and households wishing to install renewable heat facilities [27]. Since funds are limited, there is no systematic fiscal heat incentive comparable to NFFO or the RO. The potential size of the biomass heat market in 2010, assumed to consist of all residential, commercial and industrial premises not supplied by the national gas grid, is over 653 PJ per year according to FES [28]. However, the same study’s actual projection of market demand, taking into account space constraints and the speed at which boilers could be installed, falls to 15 PJ per year. According to the Biomass Strategy Report [19], support of £20/MWh (h28.92) would be required to make biomass pellets competitive with fuel oil in the domestic heat market while logs could be competitive without support, but only in a scenario where biomass prices are low and fossil fuel prices high. According to the same analysis [19] biomass boilers typically have higher capital costs and lower fuel costs compared to heating systems that use fuel oil. This means that biomass heat is most competitive compared to oil in markets where boilers operate at high loads.
3.4.1. Biomass CHP
In 2004, total inputs of renewable fuels into CHP were 2744 GWh, representing 2.2% of total energy inputs [1]. Most of the renewable fuels in question are waste products such as landfill gas, sewage gas and MSW. Though biomass CHP schemes are relatively unattractive compared to biomass heat-only facilities because of the additional cost of capital [28], the competitiveness of CHP is aided by the ability to gain ROCs from the electricity generated [6]. At present, there are a number of biomass CHP plants operating in the UK, though their aggregate contribution to the energy sector is small. The majority of CHP fuel consumption in the UK takes place in the 9 These are UK regulations that transpose the European Waste Incineration Directive, 2000/76/EC. industrial sector (chemicals, oil refineries and pulp and paper account for over 70%) while 7% is used in the electricity supply industry [1]. This is because for optimal operations, a CHP plant needs a steady source of demand for the heat output [19]. #p#分页标题#e#
3.5. Biofuels for transport
Like all Member States of the European Union, the UK is subject to the Biofuels Directive [29] and is required to set a minimum target for the penetration of biofuels into the transport fuel market. Policies to encourage biofuel penetration include reduced fuel duty for biofuels and grant support for development of refining and processing infrastructure. In 2005, total biofuel sales accounted for 0.18% of total fuel sales by energy content [30]. The UK has set a target that biofuels should reach 5% of fuel sales by volume10 in 2010/11, and 10% by 2015. The road transport fuel market in the UK has seen increasing dieselisation since 1999 with falling petrol sales and rising diesel sales in the transport sector [1]. If this pattern continues, the 5% Renewable Transport Fuel Obligation (RTFO) target would require around 1 billion litres each of biodiesel and bioethanol, 38.2 and 21.9 PJ, respectively, to be made available.11 A 5% volume blend is the maximum that a fuel is permitted to have in order to comply with fuel standards EN 590 for diesel and EN 228 for petrol. It is therefore the maximum market penetration that can be achieved by blending biofuel in unmodified vehicles but is below the Biofuels Directive reference target of 5.75% by energy content. However, the European Commission has proposed to alter the fuel standards to allow 10% biofuel blends in petrol and diesel [32]. This would allow the target to be met without relying on the development of high-blend markets. The UK target will be supported by a RTFO that will apply to fuel suppliers at the aggregate level, and will not separate targets for biodiesel and bioethanol. It is expected that RTFO will include a system of cap-and-trade permits similar to the RO in the electricity market and incorporate a requirement to report on carbon savings and against sustainability indicators [33]. Though in future the RTFO and fuel duty incentive may apply to a number of biofuels (such as biogas or pure plant oil), these policies currently apply only to bioethanol and biodiesel. Blended petrol, containing bioethanol derived from Brazilian sugar cane, is sold by one national retailer in the UK12 while 33 million litres of biodiesel were sold in 2005 [30]. 10 Equivalent to 3% bioethanol and 4% biodiesel by energy content. Energy content of fuels per litre taken from European Commission [31]. 11 Assumes same linear growth from 2007 to 2010 as per 1998–2006. Projected 2010 demand of 16 494 394 tonne petrol and 22 459 282 tonne diesel converted to energy equivalent and
BIOMASS AND BIOENERGY 32 (2008) 688– 701
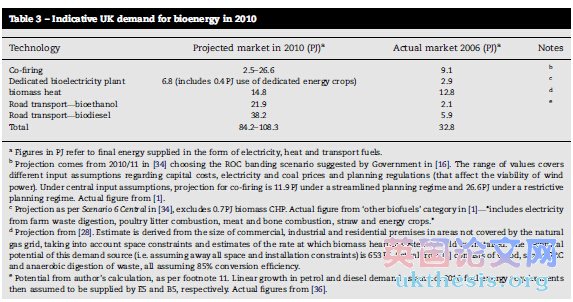
Table 3 Indicative UK demand for bioenergy in 2010 Technology Projected market in 2010 (PJ)a Actual market 2006 (PJ)a Notes Co-firing 2.5–26.6 9.1 b Dedicated bioelectricity plant 6.8 (includes 0.4 PJ use of dedicated energy crops) 2.9 biomass heat 14.8 12.8 d Road transport—bioethanol 21.9 2.1 e Road transport—biodiesel 38.2 5.9 Total 84.2–108.3 32.8 a Figures in PJ refer to final energy supplied in the form of electricity, heat and transport fuels. b Projection comes from 2010/11 in [34] choosing the ROC banding scenario suggested by Government in [16]. The range of values covers different input assumptions regarding capital costs, electricity and coal prices and planning regulations (that affect the viability of wind power). Under central input assumptions, projection for co-firing is 11.9 PJ under a streamlined planning regime and 26.6 PJ under a restrictive planning regime. Actual figure from [1]. c Projection as per Scenario 6 Central in [34], excludes 0.7 PJ biomass CHP. Actual figure from ‘other biofuels’ category in [1]—‘‘includes electricity from farm waste digestion, poultry litter combustion, meat and bone combustion, straw and energy crops.’’ d Projection from [28]. Estimate is derived from the size of commercial, industrial and residential premises in areas not covered by the natural gas grid, taking into account space constraints and estimates of the rate at which biomass heating systems could be installed. The technical potential of this demand source (i.e. assuming away all space and installation constraints) is 653 PJ. Actual from [1] consists of wood, straw, SRC and anaerobic digestion of waste, all assuming 85% conversion efficiency. e Potential from author’s calculation, as per footnote 11. Linear growth in petrol and diesel demand assumed. 2010 fuel energy requirements then assumed to be supplied by E5 and B5, respectively. Actual figures from [36]. #p#分页标题#e#
3.6. Summary of projected UK bioenergy demand for 2010
As the analysis in Section 3 has shown, UK demand for bioenergy in the near future will depend largely on the renewable fuel obligation policies in transport and electricity markets. Under the RO, biomass-derived electricity is supported but must compete against other RE options. Given the lack of specific renewable heat policy, potential biomass schemes in the heat market also have to compete against alternative heat and micro-generation technologies for limited grant funding.13 By contrast, the transport sector has a specific obligation to blend bioethanol and/or biodiesel into the road transport fuel sold. Given that the RO is broadly technology-neutral (although it will become less so if banded), predictions of the role of biomass in future energy scenarios are inherently uncertain. Table 3 gives an estimate of the size of the UK bioenergy market, in the presence of competing sources of both renewable and fossil energy, obtained by combining demand projections for the year 2010 from Oxera [34], FES [28] and DTI [1]. The biomass demanded in Table 3 equates to an input requirement for 47–116 PJ of biomass for heat and power.14 Assuming wheat and rapeseed are used as inputs for bioethanol and biodiesel, respectively, meeting the 2010 RTFO target would require the availability of 2.8 Mt of each crop.15 Taking this level of demand as an indication of bioenergy requirement, Section 4 explores the issues surrounding the role of imported biomass in the UK energy sector. 13 See [19]. 15 Fresh weight of wheat and rapeseed. 1 tonne wheat . 362 l ethanol, 1 tonne rapeseed . 422 l biodiesel. Conversion factors taken from [35].
4. Domestic and imported bioenergy feedstocks 国内和进口生物能源原料
This section investigates how the near-term demand for bioenergy, as characterised in Section 3.6, could be met. Section 4.1 provides an assessment of the quantities of biomass that could be supplied to UK markets in the short term and provides an overview of the factors that may prevent the seemingly substantial domestic resource potential from being fully utilised. The failure to provide sufficient competitively priced biomass from domestic sources provides an opportunity for bioenergy trade. Section 4.2 gives an overview of UK bioenergy trade as it has developed in recent years.
4.1. Domestic sources of bioenergy
In order to satisfy the demand for heat and power presented in Table 3, a biomass resource of 47–116 PJ would be required.
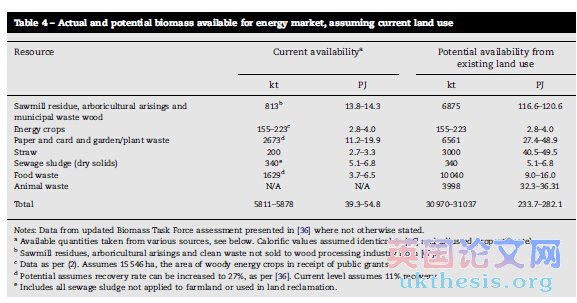
Table 4 gives an illustration of the potential contribution of domestic biomass resources to this demand. ‘‘Potential’’ in this case refers to the maximum amount of biomass that could be made available to energy markets, given the current land-use pattern in the UK and the demands from other sectors (resources that would be recycled or used by the wood processing industry are excluded). The table shows that full mobilisation of all currently available biomass could supply around half of the projected biomass demand and that these resources are underexploited at present. However, the assessment in Table 4 does not take account of the marginal cost of exploiting large proportions of the identified biomass resources or the ability of these resources to compete in energy markets with imported biomass or other energy sources. #p#分页标题#e#
BIOMASS AND BIOENERGY 32 (2008) 688– 701 Table 4 Actual and potential biomass available for energy market, assuming current land use Resource Current availabilitya Potential availability from existing land use kt PJ kt PJ Sawmill residue, arboricultural arisings and municipal waste wood Energy crops Paper and card and garden/plant waste Straw Sewage sludge (dry solids) Food waste Animal waste 813b 155–223c 2673d 200 340e 1629d N/A 13.8–14.3 2.8–4.0 11.2–19.9 2.7–3.3 5.1–6.8 3.7–6.5 N/A 6875 155–223 6561 3000 340 10 040 3998 116.6–120.6 2.8–4.0 27.4–48.9 40.5–49.5 5.1–6.8 9.0–16.0 32.3–36.31 Total 5811–5878 39.3–54.8 30 970–31 037 233.7–282.1 Notes: Data from updated Biomass Task Force assessment presented in [36] where not otherwise stated. a Available quantities taken from various sources, see below. Calorific values assumed identical to [36] and adjusted proportionately. b Sawmill residues, arboricultural arisings and clean waste not sold to wood processing industry from [67]. c Data as per (2). Assumes 15 546 ha, the area of woody energy crops in receipt of public grants. d Potential assumes recovery rate can be increased to 27%, as per [36]. Current level assumes 11% recovery. e Includes all sewage sludge not applied to farmland or used in land reclamation. Longer-term assessments, that allow for changes in land use, estimate greater biomass availability. The UK Biomass Strategy [36], gives a technical potential for primary energy of 346 PJ. This estimate includes the exploitation of 3 Mt of cereal straw and the provision of 62 PJ from 350 000 ha of woody energy crops. A separate study by the Carbon Trust [37], based on identification of the most profitable supply chains, found that 148 PJ of biomass could potentially be supplied, increasing to 288 PJ if an area of 680 000 ha were planted with woody energy crops. The European Environment Agency (EEA) [38] estimated the potential biomass production for the UK in 2010 to be 565 PJ, of which 142 PJ could come from agriculture, assuming the availability of 824 000 ha of arable land. A further 360 PJ of this potential is estimated to come from waste, predominantly the organic fraction of MSW. To assess the potential for production of liquid biofuels from domestic feedstocks, the Biomass Strategy [36] assumes that around 1.7 Mt of both wheat (for bioethanol) and oilseed rape (for biodiesel) can be grown in the UK. This is estimated to represent 50% of the 5% blend required by the RTFO, the rationale being that the rest will be supplied by imports and domestic production may increase if and when second generation technologies allow biofuels to be produced from a smaller land area. A comparison between this requirement and current UK production of wheat and oilseed rape is shown in Table 5. As Table 5 shows, the UK theoretically produces sufficient quantities of both wheat and oilseed rape to meet the full requirement of the RTFO. Ruling out the use of commercial crops for biofuel production, most of the 50% target for biodiesel could be met were land currently used as setaside to be devoted to the cultivation of oilseed rape (not that this is likely given the 0% setaside rate for to be introduced for 2007/ 08 planting). Domestic biofuel feedstocks may also come from crops currently grown on arable land and sold to commercial markets and from expansion of the cultivated area. Mattison and Norris [39] undertook a survey of UK farmers and found that 83.81% and 69.06% were either undecided or intended to grow sugar beet or oilseed rape, respectively for biofuel.16 The authors speculate that applying this willingness to supply to energy markets across the current producers of these crops could result in production of around 1.5% and 4% of UK 2010 petrol and diesel energy requirements, respectively. Whether or not the projection itself is accurate, it does demonstrate UK farmers’ willingness to grow commercial arable crops for bioenergy should the market be favourable. DEFRA [36] estimate that 740 000 ha would be needed to grow the assumed 50% of domestic biofuel and that this is below the 824 000 ha of land identified by EEA [38] as available under sustainable (and non-food-competing) conditions. However, neither estimate takes account of the cost or ease of citing the additional cultivation while the DEFRA assessment assumes that existing yields will be maintained. This is not necessarily a reasonable assumption since yields for oilseed rape grown on setaside land in the UK are 20% lower than yields on non-setaside land [40]. A number of biofuel production facilities designed for the processing of arable crops exist or are under construction in the UK. The largest proposed plants are located close to ports, implying an important role for imported feedstocks.17 Biodiesel can also be produced from waste animal fats and vegetable oils, as at the Argent Energy plant in Scotland.18 If liquid biofuels are produced on a large scale in the UK (or indeed elsewhere), the cultivation would also produce significant quantities of residues suitable for combustion in 16 Indicates percentage of respondents who indicated they were either undecided, intending or strongly intending to grow the crop for bioenergy markets. 17 For example see BIOMASS AND BIOENERGY 32 (2008) 688– 701 Table 5 Potential biofuel feedstocks from existing land use Resource Current availability (Mt) Potential no-foodcompetition availability from existing land use (Mt) Current UK production (on all land types) (Mt) UK biofuel feedstock cultivation Oilseed rape Wheat Sugar beet 0.51a N/A N/A 0.74–1.6b 1.01c N/A 1.87 14.74 7.15 UK feedstock requirementd Oilseed rape Wheat 1.68 1.72 Source: Agriculture in the United Kingdom, 2006 [40] unless otherwise stated. a Oilseed rape currently grown on setaside land plus rape receiving support from Energy Crop Aid Scheme (2005 figure) [68]. b As per (1) but cultivation expanded to cover whole setaside area at same yield. Lower figure assumes 350 000 ha of setaside are used for woody energy crops (see Table 3). c Figure is 2006 net export of wheat. d Figure from DEFRA Biomass Strategy. Assumes that half of the 2010 RTFO requirement is to be met by domestic wheat and oilseed rape. stationary facilities, such as rapemeal from oilseed rape or DDGS (distiller’s dried grains with solubles) from wheat. This material could boost the resources identified in Table 4 as available for heat and power generation. For example, use of the 2006 net wheat surplus (1.01 Mt [40]) for the production of bioethanol would result in the co-production of around 446 500 tonnes of dried distillers grains (DDGS)19 [35] while the cultivation of oilseed rape on 255 727 ha20 (a combination of setaside and arable land) would produce 397 000 tonnes of rapemeal21 [35]. The combined energy content of these co-products would be 14.5 PJ.22 Products such as DDGS and rapemeal are currently consumed and traded internationally as protein-rich animal feeds. As a combustion feedstock, these residues are attractive due to their low moisture content, low processing need compared to forestry waste and potential to receive extra support under the RO if the crop as a whole meets the criteria for classification as a purposely grown energy crop [16,44]. It should be noted, however, that increased production of these by-products, at a time of rising global demand for animal feed, does not guarantee their availability as a competitively priced bioenergy feedstock. #p#分页标题#e#
4.2. Bioenergy imports
Through a substantial resource flow, the actual amount of biomass currently imported for energy is difficult to estimate 19 Assumes 362 l of bioethanol per fresh tonne of wheat (16% moisture), 1.2 kg of DDGS per litre of bioethanol as per Smeets et al. [35]. Official UK statistics report wheat with 14.5% moisture content. 20 Assumes current energy crop aid scheme production (315,000 tonne) plus 163 000 ha of setaside (with yield of 2.6 tonne/ha). Leaves 350 000 ha of setaside for woody energy crops. 21 Assumes 0.59 tonne rapemeal per tonne of dry rapeseed as per [41]. Official UK statistics report rapeseed with 9% moisture content. 22 Assumes net calorific value of 16.056 MJ/kg for rapemeal as per [42] and 18.2 MJ/kg for DDGS as per [43]. due to scarcity of data. It is particularly difficult to identify which biomass flows are intended for eventual energy use. This section combines data from various sources to identify the major bioenergy flows into the UK. In order to meet the RTFO targets for 2010, the UK is likely to import large quantities of bioethanol and vegetable oils (as biodiesel feedstocks). In the case of stationary combustion for heat and power, a wider choice of feedstocks is available, as Fig. 2 shows. This section considers the near-term availability of agricultural residues and wood pellet, the two major types of feedstock imported into the UK 2005.23 In the longer term, different feedstocks may become available with advances in logistics and biomass conversion technologies. In 2005, over 1.4 Mt of biomass was co-fired [45] using a variety of feedstocks (see Fig. 2). Over 1 Mt of this consisted of imported material, primarily palm and olive residues, tall oil and wood pellets.24 In addition to co-fired biomass, domestic sales of bioethanol in 2005 were 85 million litres (1.8 PJ) at a time when bioethanol for transport was not being produced commercially in the UK [30]. Vegetable oils are also imported into the UK for conversion into biodiesel. It is estimated that 50 000 tonnes of palm oil were imported for conversion to biodiesel in 2006 [46] and that demand for biofuels is responsible for the 76% increase in UK imports of palm, soya and rapeseed oil since 2004 [36]. In 2005, 380 388 tonnes of fuelwood25 were imported (and 236 761 tonnes exported) [47], although this is likely to include the wood pellet imported for co-firing with coal. As discussed in Section 3.3, co-firing developed quickly in the UK because of the support offered under the RO. In the 23 The only year for which detailed data are available. 24 Material assumed to be imported since biomass in question is not produced in the UK. It is possible that a fraction of the co-fired wood pellet originated in the UK since the first pellet plants opened in the year 2005. 25 CEN 4401: Fuel wood, in logs, in billets, in twigs, in faggots or in similar forms.
Olive wastes, Olive wastes, BIOMASS AND BIOENERGY 32 (2008) 688– 701 Other, 71,044 WDF and Wood, 102,034 Palm waste, 449,657 Tallow, 119,828 CCP and pellets, 102,246 Tall Oil, 120,129 Wood pellets, 163,961 283,222 #p#分页标题#e#
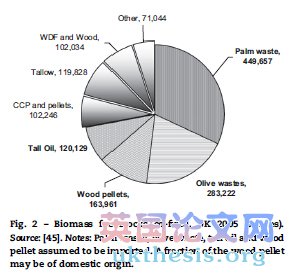
Fig. 2 – Biomass feedstocks co-fired, UK 2005 (tonnes). Source: [45]. Notes: Palm waste, olive waste, tall oil and wood pellet assumed to be imported. A fraction of the wood pellet may be of domestic origin. absence of domestic energy crops and certainty over future support levels, this framework encouraged large import volumes. In this situation, feedstocks such as PKE, olive cake and pelletised biomass, that can be bought on spot markets and co-fired with minimal modifications to existing plant, are preferable to the capital investments and supply chain infrastructure that would be needed to co-fire woody energy crops or exploit domestic resources such as forestry residue [14]. The olive cake consumed by co-firers in 2005 represents approximately 6% of the estimated 5 Mt annual world production [45]. Much of this may be unmarketed or used locally where olive oil is produced. According to Eurostat, EU countries imported 318 000 tonnes of olive cake in 2005, over half of which was dry olive cake imported into the UK [14]. Worldwide production of PKE is estimated to reach approximately 4.5 Mt26 by 2010 [48], compared to 3.2 Mt in 2005 [49]. Other palm residues, such as empty fruit bunches and shells, are also suitable for combustion. Current global production of rapemeal is approximately 28 Mt27 [41,49]. Hypothetically, cultivating additional rapeseed to satisfy the projected biodiesel demand for the EU-25 in 2010 would produce approximately 17 Mt.28 Producing a quantity of wheat-derived bioethanol equivalent to the projected EU-25 demand for 2010 would produce an additional 14.5 Mt29 of DDGS. Since DDGS is a by-product of the distillation process, increased use of cereal grains specifically for bioethanol production (or other processes involving distillation) is necessary for 26 Assumes 0.1 tonne of PKE produced per tonne of palm oil. 27 FAOSTAT gives 2005 dry rapeseed production of 47.6 Mt. Assumes 0.59 tonne rapemeal per tonne dry rapeseed as per [41]. 28 Assume 13 billion litres of biodiesel demanded as per [49], 422 l biodiesel per tonne of rapeseed (fresh) [35] and 0.55 tonne rapemeal per tonne of rapeseed (fresh) as per [41]. 29 Assume 12 billion litres of bioethanol demanded as per [50], 1255 l of bioethanol per tonne as per [31] and 3.03 tonne wheat (dry) per tonne of bioethanol and 0.5 tonne DDGS per tonne wheat (dry) as per [35]. these residues to become available. In the case of rape and palm oil, increased cultivation will lead to greater residue availability, regardless of whether the oil itself is sold to food or fuel markets. However, most by-products from transport fuel have important non-energy uses. Most importantly, PKE and DDGS are already traded internationally as animal feed. Prices of the olive and palm residues currently imported have risen in the past year due to extreme weather conditions in South-East Asia while PKE’s share of the animal feed market is growing [51]. In future, the downward pressure on prices of combustible crop residues, brought about by increased cultivation of liquid biofuel feedstocks, could be reduced or cancelled out due to increased worldwide demand for food and, in particular, meat consumption. The 2007 FAO Agricultural Outlook [48] (the first year in which the publication’s projections explicitly take account of the effects of biofuel policies) predicts that the world price of oilseed meals will remain above current levels from 2007 to 2013, peaking in 2009 at 8% above current levels, and fall thereafter. Other crops whose residues were co-fired in 2005 include shea and sunflower. The share of other crop residues in co-firing could increase in future if high-protein meals such as PKE and rapemeal become unaffordable due to competition from feed markets. However, prices for these products are also likely to increase if food and bioenergy markets expand worldwide. UK co-firers combusted 163 000 tonnes of wood pellet in 2005. It is likely that significant quantities of pellet were also imported for heating applications, although detailed statistics are not available. Wood pellets are usually formed from compressed sawdust obtained from residues in wood products industries. Advantages of using wood pellet include low bulk density and low moisture content. In addition, wood pellet is a manufactured product for which standardised specifications exist in several major markets [52]. The ability to provide a uniform product with predictable combustion properties could prove advantageous to major UK importers who will potentially import feedstocks from several different sources. Unlike cereal and oilseed residues the global wood pellet market is in the process of worldwide expansion and consumers do not face competition from non-energy markets. The largest producers of wood pellet are Sweden and Canada, although Sweden is also a substantial consumer and importer of bioenergy [53]. Two countries experiencing rapid growth in wood pellet exports are Canada and Russia. In Canada, around 1.2 Mt of pellet was produced in 2006, with production expected to reach 6 Mt by 2010 [54]. Only 1 Mt of pellet was consumed in Canada in 2006 with growth of less than 500 000 tonnes expected by 2010 [55]. Russia contains 23% of the world forest area. This forest produces 14–15 billion tonnes of biomass annually but only a fraction is utilised. North-West Russia contains 17% of the Russian forest resource, a growing stock of 15 billion m3 from which 43.7 million m3 is harvested annually. By comparison, Finland harvests 60 million m3 per year from a growing stock of 2 billion m3 [56]. Modernisation of the Russian forestry sector is in progress, with h350 million invested by Finnish firms up to 2006. Production of wood pellet in Russia is expected to reach 600 000 tonnes in 2007 [57]. #p#分页标题#e#
BIOMASS AND BIOENERGY 32 (2008) 688– 701
5. Greenhouse gas savings from bioenergy trade 生物能源行业的温室气体储蓄
In order to determine the net GHG emissions avoided through replacement of alternative energy sources with imported biomass, an assessment is required that takes into account the emissions from production, conversion and transport of the biomass as well as land-use change and displacement caused by the diversion of biomass away from other markets. Bioenergy feedstocks can usually be categorised either as energy crops or residue-based fuels. Energy crops are defined as crops where the majority of sales revenue comes from uses other than food or fodder [58] while residues are defined in studies such as Woods et al. [45] as biomass whose value does not exceed 10% of factory-gate income. This distinction is important for determining the system boundary of a bioenergy flow. If a feedstock is grown with the intention to be used as energy, the net emissions from the entire life-cycle of the crop are attributable to bioenergy use. Alternatively, in the case of residues whose value is so low that their sales potential has no effect on the decision to plant or harvest a given bioenergy source, only the net change in emissions resulting from the residue’s use as bioenergy is attributable. Thus the net emissions from combustion of sawdust that would otherwise go to landfill would include a credit for the avoided methane emissions while the use of sawdust that would otherwise be used in wood panel manufacture would include the extra emissions resulting from that industry’s extra wood purchase. Ceteris paribus, emissions from transport will be lower for residue-based feedstocks than dedicated energy crops since for residues, only transport emissions from factory gate to power plant are attributable. Although GHG embodied in transport increases with total distance travelled, emissions are substantially lower for long-distance shipping (0.012– 0.024 kgCO2eq/odt/km) than for truck transport (0.18– 0.27 kgCO2eq/odt/km) [59], implying that the embodied emissions from imported biomass combustion need not be significantly greater than from equivalent domestic sources. For co-firing, Woods et al. [45] find the life-cycle emissions from combustion of South African coal to be 939 gCO2/KWhe, of which 3.3% of emissions comes from coal transportation. This compares to 1.85 gCO2/KWhe (7.5% of life cycle emissions) and 10.8 gCO2/KWhe (1.1%) from local miscanthus and imported Swedish wood pellet,30 respectively. More significant than the emissions from transport are the potential emissions from land-use change and the allocation of emissions between products, co-products and residues. In the case of land-use change, the loss of above-ground biomass and depletion of soil carbon levels can substantially outweigh the emissions avoided through fossil fuel savings. This is illustrated in Reinhardt et al. [60] where life cycle GHG emissions from palm oil-derived biodiesel and fossil-derived diesel are compared. Biodiesel grown on land that was previously natural forest was found to reduce emissions only when losses from land-use change are depreciated over a 100-year period. By contrast, palm oil grown on fallow land provides emissions savings even when depreciated over a 25 year period. Wood pellet combustion in Woods et al. [45] provides a good illustration of the importance of residue allocation. Emissions from transport, manufacture and combustion of imported wood pellet are greater than coal at 967 gCO2/KWhe. Assuming that the sawdust used to make the wood pellet would otherwise have been sent to landfill, these emissions are outweighed by a 4215 gCO2/kg credit for CO2 and methane emissions avoided [61]. Removing the landfill credit but cancelling out the direct CO2 emissions from pellet combustion, on the assumption that the forest is re-planted and hence absorbs CO2, reduces emissions by 895 g to 72 gCO2/KWhe,31 compared to around 20 gCO2/KWhe for SRC and miscanthus. However, when the bioenergy market purchases biomass residues that would otherwise have been sold elsewhere, the GHG emissions embodied in the manufacture of a substitute product need to be counted. In the case of co-firing imported palm kernel shell to displace coal, Damen and Faaij [62] find that the emissions savings are roughly 30% lower when the shell would have been used for animal feed compared to the case where shells would have been burned in the open air. Indirect emissions, either from land-use change or displacement of co-product markets add a further complication to the issue of GHG balance calculation, as noted by Cramer et al. [63]. This can occur when biomass that would otherwise have been consumed elsewhere is diverted to energy markets, leading to the production of a substitute for the non-energy product that itself contains embodied GHG emissions. In Reinhardt [60], the GHG balance of palm oil biodiesel worsens substantially when palm oil (for cooking) or rubber is replaced, instead of natural forest. This is because the GHG balance in these cases includes the emissions embodied in the production of vegetable oil or synthetic rubber to replace the non-energy product. Data requirements for assessing the GHG balance of a bioenergy chain are therefore considerable. Necessary information would include knowledge of prior land use, production, processing and transport practices as well as the relative value of each element of the total crop. In the UK, a carbon certification system is being developed under the RTFO [64] that will collect data on previous land-use and co-product allocation for liquid biofuel chains.32 The scheme will not initially provide extra reward to biofuels that demonstrate the greatest emissions savings but will publish the carbon performance of each fuel supplier. The reporting scheme will include ‘‘conservative’’ default values designed to encourage suppliers to report the greatest possible detail concerning their fuels’ origin. No policy is yet proposed to monitor the GHG balance of biomass feedstocks used for electricity generation under the RO. This could have important implications for the GHG balance of UK bioenergy trade if growth in feed markets leads to more intense competition for residues between energy and agricultural markets, invalidating the 30 The wood pellet calculation does not take into account the 31 Assumes CH4 and NOx emissions are 20 g CO2/kg. landfill methane avoided. A substantial credit for this is provided 32 Similar schemes are under development in Germany and in [45]. the Netherlands; see [65,63]. #p#分页标题#e#
BIOMASS AND BIOENERGY 32 (2008) 688– 701 assumption that feedstocks would otherwise have been disposed of as waste.
6. Discussion 讨论
Sections 1–5 have highlighted several characteristics of the UK energy sector which are unlikely to change in the near future. The electricity supply relies on centralised generation and long-distance distribution, as opposed to local generation and distribution. This situation is reinforced by the planning regime and the RO that rewards electricity generation to the disadvantage of heat and CHP. At the same time, the wide coverage of the national gas grid reduces the opportunity for biomass heating systems to compete in domestic and light commercial markets. By co-firing 10% biomass in existing coal plants, instead of the 2.26% co-fired in 2006, the UK could produce in excess of 39 PJ of electricity, more than the total electricity and heat produced from biomass in 2006. In less than 5 years, generation from co-firing has reached 9 PJ, in spite of a policy framework that places greater restrictions and uncertainty on co-firing compared to other renewable technologies. In contrast, the recent history of dedicated bioelectricity plants has been one of numerous policy initiatives that have not resulted in substantial levels of electricity generation. Less than half the NFFO biomass contracts remained active in 2003, and none of the advanced technology contracts have been commissioned. This situation may be improving, however, with two large wood-fired plants commissioned in 2007. It is anticipated that in future a banded ROC tariff will replace the current RO framework, including a removal of the cap on co-firing support. Depending on the size of the RO and the relative bands awarded to different technologies, banding could encourage growth in both co-firing and dedicated bioelectricity generation. The market for transport biofuels is also expected to grow to over 60 PJ as a result of the RTFO. Grant support and the availability of convenient products such as wood pellet could lead to substantial growth in the biomass heat market. However, there is no systematic incentive comparable to the RO or RTFO in the heat market. The coverage provided by the national gas grid is also a barrier.
Table 4 shows that approximately 30 PJ of primary energy, a substantial fraction of the biomass that would be required for UK heat and electricity markets, could be supplied through domestic sources given existing land use. However, this would require substantial logistical mobilisation, high levels of waste recovery and an energy infrastructure capable of using a variety of feedstock types. These resources could be supplemented by the cultivation of liquid biofuel feedstocks (and the combustion of their residues) or woody energy crops. According to the most optimistic estimate [38], 824 000 ha of arable land could be available for energy crop cultivation, producing 565 PJ of bioenergy feedstocks, without competing with food production. For transport fuel, sufficient wheat, oilseed rape and sugar beet could be produced to meet half the UK’s 2010 biofuel requirement. However, using these crops for biofuels, especially in the case of oilseed rape, would require the diversion of significant quantities of the crop from existing markets. Given the attractiveness of wheat, oilseed rape and agricultural residues to non-energy markets and the extent to which domestic resources would have to be exploited in order to satisfy demand, it seems likely that large quantities of biomass will continue to be imported into the UK for energy. This appears most likely for transport fuels, where both feedstocks and final products are available on international markets and a substantial market is guaranteed by the RTFO. In the case of feedstocks for heat and power, the future extent of UK bioenergy trade is less certain. This is because in the electricity market, biomass technologies compete for support with other renewable technologies and, in the case of co-firing, the option always exists for operators of coal plants to decrease the proportion of biomass fired and produce or purchase ROCs from other technologies. Due to the relatively low GHG emissions per km for biomass transported internationally, it does not appear that imported biomass per se should be discouraged on the grounds of emissions savings. However, a great detail of information would be required in order to accurately calculate the GHG balance, and hence avoided emissions, from bioenergy. This is a wider issue, of which the questions of co-product allocation and land use discussed in this paper form an important part. If successful, the reporting mechanism and system of default values in the RTFO, and similar schemes in other countries, will encourage both more detailed reporting of the origins of transport biofuels and use of the least polluting feedstocks and processes by UK suppliers. For the UK, a similar scheme is required to assure that GHG savings are achieved through use of biomass for electricity. This is especially important as demand from both food and energy markets increases, meaning that any given source of biomass is likely to constitute a marketable co-product rather than a residue that would have been thrown away. However, it is also possible that the information required for carbon reporting under the RTFO, by including information about co-product markets, will increase the availability of information regarding the GHG balance of heat and power feedstocks. #p#分页标题#e#
7. Conclusion 总结
From the above analysis, it is clear that government incentives have been the principal driver of bioenergy use in the UK. In terms of biomass volume, the RO has been more successful than previous policies due to its inclusion of co-firing, which consumed 35 PJ of biomass in 2005 and 2006, over 30% more than the combined use of wood, straw and energy crops in heat and electricity production in dedicated biomass plants. By 2010, the UK could require up to 48.2 PJ of heat and power from biomass, equivalent to input energy of 116 PJ, and a further 60 PJ of bioethanol or biodiesel. A large fraction of this demand could potentially be met by biomass from domestic sources, with 39–230 PJ potentially available from residue and waste streams and 62–565 PJ attainable through mass cultivation of energy crops. However, realisation of this potential
BIOMASS AND BIOENERGY 32 (2008) 688– 701 depends upon hypothetical increases in waste and residue recovery rates and changes in land use. To date a large fraction of UK bioenergy has derived from imported biomass, particularly in the case of co-firing and bioethanol. Further research is needed to determine whether future bioenergy demand will make greater use of domestic resources. This will depend largely on the ability of policies such as the RO and RTFO to create stable long-term bioenergy markets that merit investment in collection and processing infrastructure. Such a development appears most likely in transport markets, where the available resources (primarily wheat and oilseed rape) are already produced and sold to other markets. For heat and power, investment is required to convert identified resources into bioenergy. The RO, as a framework in which different technologies compete for support, may not be the ideal tool to promote such investment, although banding may improve this situation. Despite uncertainty over the size and stability of the biomass market in heat and power, the UK represents a potentially large market for imported biomass, even without significant investment in bioenergy infrastructure. The expected demand for liquid biofuels in 2010 is several times greater than the potential production from industrial rapeseed and net wheat exports. In addition, were UK coal plants to achieve 10% co-firing, around 155 PJ of biomass would be needed. The extent to which UK bioenergy trade leads to GHG mitigation remains to be seen and depends on many factors. However, the development of RTFO carbon reporting, which could eventually lead to minimum GHG standards for biofuels, provides an important first step in assuring the carbon mitigation of bioenergy on a large scale.
REFERENCES 文献
[1] DTI. Digest of United Kingdom energy statistics. Department of Trade and Industry; 2006. [2] OFGEM, DTI. A call for evidence for the review of barriers and incentives to distributed electricity generation, including combined heat and power. Department of Trade and Industry; 2006. [3] Tovey N. The changing face of the electricity markets in the UK. [4] OFGEM. OFGEM’s response to the BERR consultation on reform of the renewables obligation. OFGEM; 2007. [5] DTI. The energy challenge. Energy review. A report. Department of Trade and Industry; 2006. [6] Non Fossil Purchasing Agency N. Role of NFPA (Accessed 18/12/2006), 2006. HM Revenue and Customs; 2006. [8] Energy Business Review. Volatility the only certainty in EU carbon market. Energy Business Review, 8 May 2006. [9] van der Horst D. UK biomass energy since 1990: the mismatch between project types and policy objectives. Energy Policy 2005;33(5):705–16. [10] DTI, Frontier Economics, Cleirigh BO. Evaluation of DTI support for new and renewable energy under NFFO and the supporting programme. Department of Trade and Industry; 2000. [11] Boyle S, Brown P. Farmers burned as green energy plant faces export. The Guardian 31 May 2003. [12] DTI. Best practice brochure: co-firing of biomass (main report). Department of Trade and Industry; 2005. [13] NFPA. Press release: e-ROC auction of renewable obligation certificates by NFPAS-results. Non-Fossil Purchasing Agency; 2006. [14] Perry M, Rosillo-Calle F. Co-firing report—United Kingdom. IEA Task 40, 2006. [15] Ernst & Young DTI. Impact of banding the renewables obligation-costs of electricity production. DTI; 2007. [16] DTI. Reform of the renewables obligation. Department of Trade and Industry; 2007. [17] OFGEM. Generators accredited for the Renewables Obligation (RO), Renewables Obligation (Scotland) (ROS) and Northern Ireland (NIROC) with a DNC over 50 kW. OFGEM; 2007. [18] RWEnpower. RWE npower statement regarding future use of Palm Oil. RWEnpower; 2006. [19] DTI. UK biomass strategy 2007. Working Paper 1—Economic Analysis of Biomass Energy. Department of Trade and Industry; 2007. [20] Directive 2001/80/EC of the European Parliament and of the Council of 23 October 2001 on the limitation of emissions of certain pollutants into the air from large combustion plants. Official Journal of the European Communities L309. 27/11/ 2001. 1-21 [21] DTI, Irons R, Riley G, Williamson J, Wigley F, Livingston W. Low cost co-utilisation of biomass. Department of Trade and Industry; 2005. [22] DTI. IPA energy consulting, and mitsui babcock. The economics of co-firing. London: Department of Trade and Industry; 2006. [23] RWE Power International, Beeley T, Jones C, Myers A, Riley G. Direct injection the Aberthaw Story. Presentation to Renewable Energy Association Bioenergy Conference, September 2006. [24] Tillman DA. Biomass cofiring: the technology, the experience, the combustion consequences. Biomass and Bioenergy 2000;19(6):365–84. [25] DEFRA. Guidance on Directive 2000/76/EC on the incineration of waste. 3rd ed. London: Department for Environment, Food and Rural Affairs; 2006. [26] REA. The Government’s Energy Review: submission by Renewable Energy Association. Renewable Energy Association; 2006. [27] DTI. Our energy challenge: power from the people. Micro-generation strategy. Department of Trade and Industry; 2006. [28] Future Energy Solutions. Renewable heat and heat from combined heat and power plants-study and analysis. Future Energy Solutions; 2005. [29] Directive 2003/30/EC of the European Parliament and of the Council of 8 May 2003 on the promotion of the use of biofuels or other renewable fuels for transport. 08/05/2003, p. [30] DfT. Promotion and use of biofuels in the United Kingdom: UK report to European Commission under Article 4 of the Biofuels Directive (2003/30/EC), Department for Transport, 2006. [31] European Commission. Review of EU biofuels directive: public consultation exercise, April–July 2006. European Commission; 2006. [32] European Commission. Biofuel issues in the new legislation on the promotion of renewable energy. Public consultation exercise, April–May 2007. European Commission; 2007. [33] E4tech, The Edinburgh Centre for Carbon Management Ltd., Imperial Centre for Energy Policy and Technology, Bauen A, Howes J, Chase A, et al. Feasibility Study on Certification for a Renewable Transport Fuel Obligation, Department for Transport, 2005. #p#分页标题#e#
BIOMASS AND BIOENERGY 32 (2008) 688– 701 [34] Oxera. Reform of the renewables obligation: what is the likely impact of changes? Department of Trade and Industry; 2007. [35] Smeets E, Juninger M, Faaij A. Supportive study for the OECD on alternative developments in biofuel production across the world. Organisation for Economic Cooperation and Development; 2005. [36] DEFRA, DTI, DfT. UK biomass strategy. Department for Environment, Food and Rural Affairs; 2007. [37] Carbon Trust. Biomass sector review for the carbon trust. Carbon Trust; 2005. [38] European Environment Agency. How much bioenergy can Europe produce without harming the environment. Office for Official Publication of the European Communities; 2006. [39] Mattison EHA, Norris K. Intentions of UK farmers toward biofuel crop production: implications for policy targets and land use change. Environmental Science and Technology 2007;41(16):5589–94. [40] DEFRA. Agriculture in the United Kingdom: 2006. Department for Environment, Food and Rural Affairs; 2007. [41] Elsayed MA, Matthews R, Mortimer ND. Carbon and energy balances for a range of biofuels options. Resources Research Unit, Sheffield Hallam University; 2003. [42] Mortimer ND, Elsayed MA. North east biofuel supply chain carbon intensity assessment. North Energy Associates Ltd; 2006. [43] Woods J, Brown G, Estrin A. Bioethanol greenhouse gas calculator. Home-grown Cereals Authority; 2005. [44] OFGEM. Renewables obligation: fuel measurement and sampling guidance. OFGEM; 2007. [45] Woods J, Tipper R, Brown G, Diaz-Chavez R, Lovell J, Groot Pd. Evaluating the sustainability of co-firing in the UK, 2006. [46] Heap T. Biofuels—as green as they seem? In: Costing the Earth. BBC Radio 4. 17/05/2007. [47] Eurostat, 2007. EU27 Trade since 1995 by CN8. Eurostat Database [48] FAO, OECD. OECD-FAO agricultural outlook 2007–2016. Organisation for Economic Cooperation and Development; 2007. [49] FAO. FAOSTAT. Food and Agriculture Organization of the United Nations, 2007. [50] European Commission and National Technical University of Athens. European energy and transport trends to 2030. European Commission, Directorate General for Energy and Transport; 2003. [51] Biopact. Substitution logic: expensive corn creates market for oil palm protein as animal feed. Biopact.com, 2007. [52] Hahn B. Existing guidelines and quality assurance for fuel pellets. Pellets for Europe, 2004. [53] Ericsson K, Nilsson LJ. International biofuel trade—a study of the Swedish import. Biomass and Bioenergy 2004;26(3):205–20. [54] Swaan J. North American wood pellet industry update. Presentation to Bioenergy Days Conference, Lidkoping, Sweden, 24 August 2006. [55] Swaan J. ‘‘Biomass,’’ responsible, sustainable, renewable energy option. In: Presentation to conference Maximizing energy efficiency and renewable energy in British Columbia, 23 March 2006. [56] Rakitova O. Challenges of developing bioenergy markets in Russia. In: Presentation to Developing bioenergy markets—focus on forest sector in Finland and Russia, Lappeenranta, Finland, 24/10/2006. [57] Sundman S. Emerging bioenergy markets and russia: future challenges and opportunities for forest industry. In: Presentation to Developing bioenergy markets-focus on forest sector in Finland and Russia, Lappeenranta, Finland, 24/10/ 2006. [58] DEFRA, Rural Payments Agency. Set-aside handbook and guidance for England. Department of Environment, Food and Rural Affairs; 2006. [59] Royal Commission on Environmental Pollution. Biomass as a renewable energy source. Royal Commission on Environmental Pollution; 2004. [60] Reinhardt GA, Rettenmaier N, Ga¨rtner S, Pastowski A. Rain forest for biodiesel? Ecological effects of using palm oil as a source of energy. WWF Germany; 2007. [61] Mann M. A life cycle assessment of biomass cofiring in a coal-fired power plant. Clean products and processes 2004, volume 3, number 2, August 2001, p. 81–91. [62] Damen K, Faaij A. A greenhouse gas balance of two existing international biomass import chains. Mitigation and Adaptation Strategies for Global Change 2006;11(5):023–1050. [63] Cramer J, Wissema E, Lammers E, Dijk D. Criteria for sustainable biomass production. Project Group ‘Sustainable Production of Biomass’, 2006. [64] E4tech. Carbon reporting within the renewable transport fuel obligation—methodology. Department for Transport; 2007. [65] Fritsche UR, Hunecke K, Hermann A, Schulze F. Sustainability standards for bioenergy. Oeko Institut; 2006. [66] DTI. Digest of United Kingdom Energy Statistics (DUKES 2007), 2007. [67] DTI, Forestry Contracting Association, and Forestry Commission. Woodfuel Resource in Britain: main report, Department of Trade and Industry, 2003. [68] DEFRA and DTI. Creating value from renewable materials: a strategy for non-food crops and uses, two-year progress report, Department for Environment, Food and Rural Affairs, 2006. #p#分页标题#e#